Civil Engineering
The Sinking Cities of America: A Study Reveals Widespread Land Movement Across 28 Major U.S. Metropolises
A new study of the 28 most populous U.S. cities finds that all are sinking to one degree or another. The cities include not just those on the coasts, where relative sea level is a concern, but many in the interior. Furthermore, using newly granular data, the study finds that some cities are sinking at different rates in different spots, or sinking in some places and rising in others, potentially introducing stresses that could affect buildings and other infrastructure.
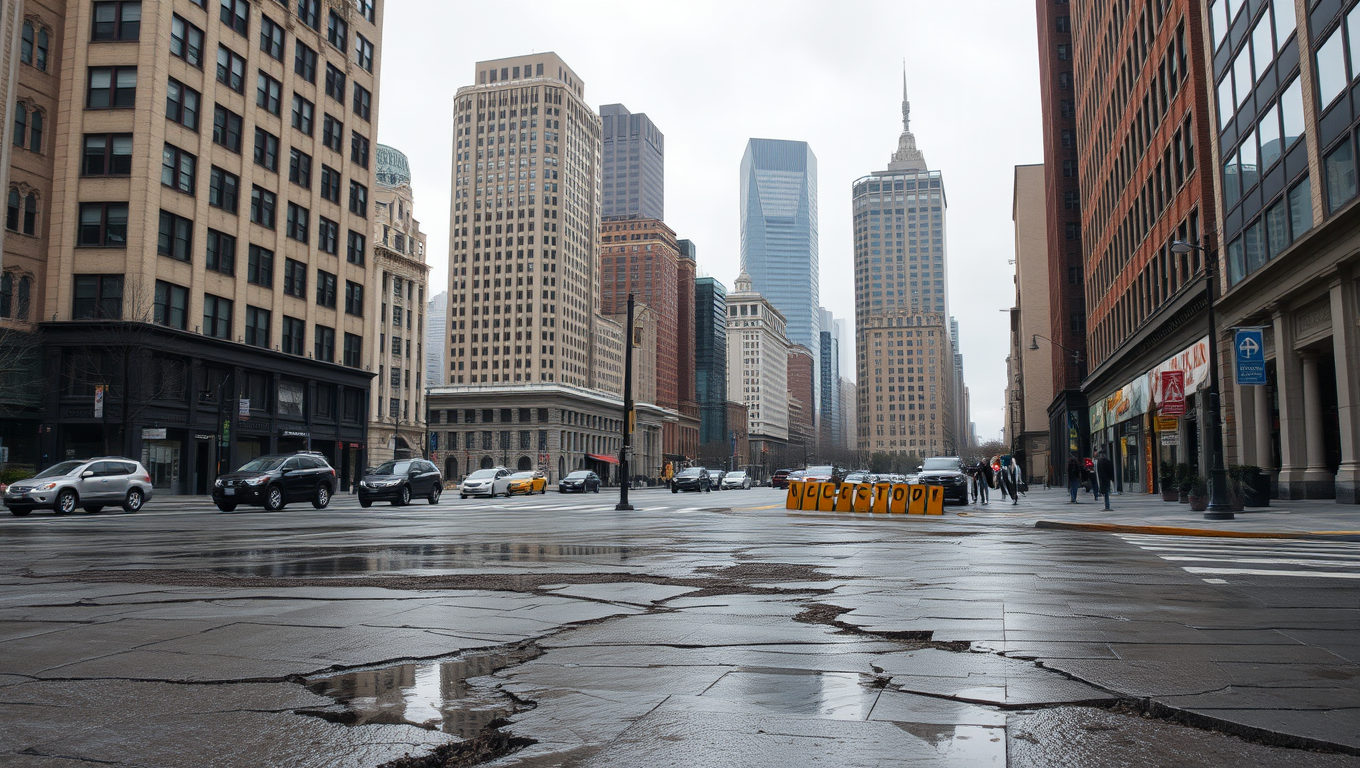
Artificial Intelligence
The Hidden Barrier to Advanced Robotic Touch
Researchers argue that the problem that has been lurking in the margins of many papers about touch sensors lies in the robotic skin itself.
Aerospace
“Challenging the Classics: Researchers Reveal New Insights into Material Deformation under Stress”
Scientists have expanded on a longstanding model governing the mechanics behind slip banding, a process that produces strain marks in metals under compression, gaining a new understanding of the behavior of advanced materials critical to energy systems, space exploration and nuclear applications.
Biochemistry
Unveiling the Mystery of Crystals: Scientists Discover a New Type and Shed Light on Their Formation
Crystals — from sugar and table salt to snowflakes and diamonds — don’t always grow in a straightforward way. Researchers have now captured this journey from amorphous blob to orderly structures. In exploring how crystals form, the researchers also came across an unusual, rod-shaped crystal that hadn’t been identified before, naming it ‘Zangenite’ for the graduate student who discovered it.
-
Detectors3 weeks ago
A New Horizon for Vision: How Gold Nanoparticles May Restore People’s Sight
-
Earth & Climate2 months ago
Retiring Abroad Can Be Lonely Business
-
Cancer2 months ago
Revolutionizing Quantum Communication: Direct Connections Between Multiple Processors
-
Agriculture and Food2 months ago
“A Sustainable Solution: Researchers Create Hybrid Cheese with 25% Pea Protein”
-
Diseases and Conditions2 months ago
Reducing Falls Among Elderly Women with Polypharmacy through Exercise Intervention
-
Albert Einstein2 months ago
Harnessing Water Waves: A Breakthrough in Controlling Floating Objects
-
Alternative Fuels2 months ago
Unlocking the Secrets of Supercritical Water: A New Understanding of Its Structure
-
Earth & Climate2 months ago
Household Electricity Three Times More Expensive Than Upcoming ‘Eco-Friendly’ Aviation E-Fuels, Study Reveals