While we try to keep things accurate, this content is part of an ongoing experiment and may not always be reliable.
Please double-check important details — we’re not responsible for how the information is used.
Cell Biology
Unlocking the Secrets of Deadly Tropical Diseases: A New Target for Treatment
The efforts of a research team give hope for new treatment approaches for dangerous tropical diseases. The researchers have compiled a high-precision inventory of the membrane proteins of cell organelles of the African sleeping sickness pathogen.
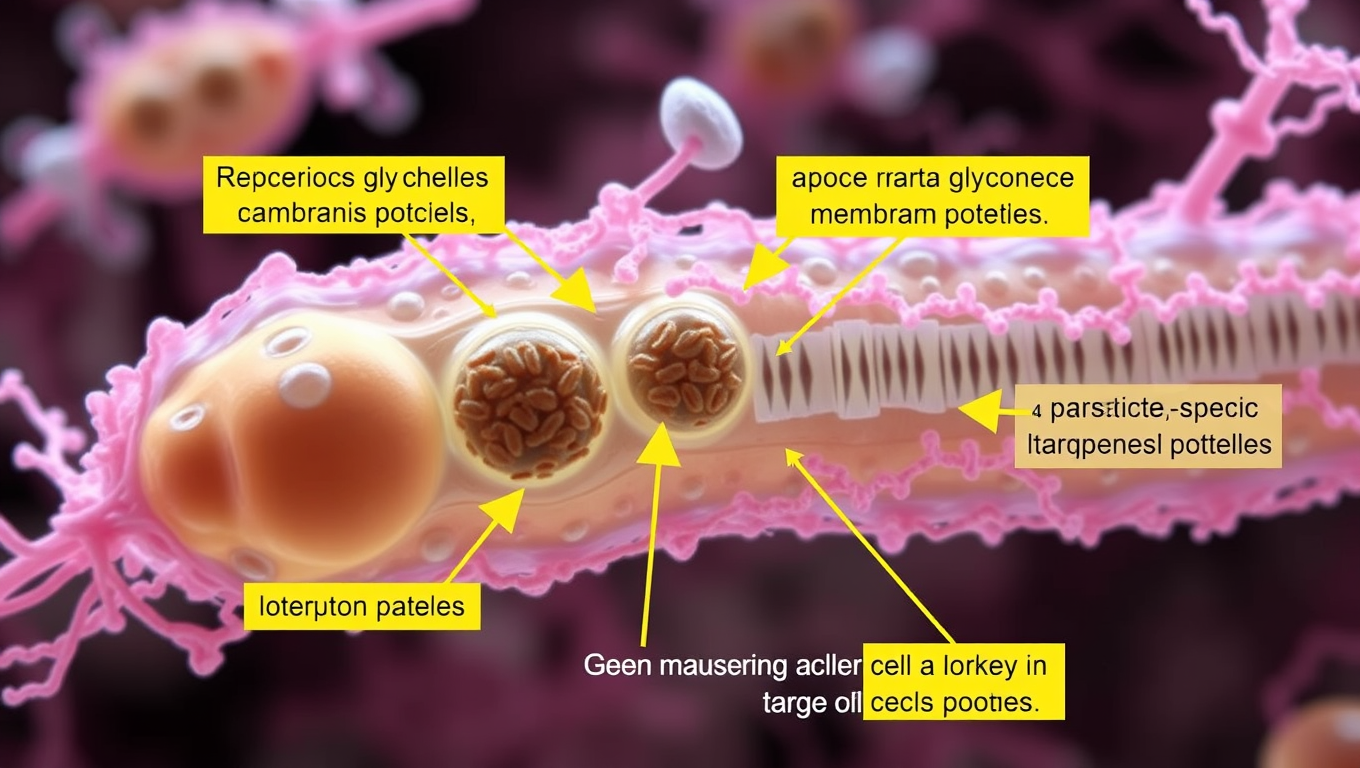
Cell Biology
A 600-Million-Year-Old Body Blueprint Uncovered in Sea Anemones
Sea anemones may hold the key to the ancient origins of body symmetry. A study from the University of Vienna shows they use a molecular mechanism known as BMP shuttling, once thought unique to bilaterally symmetrical animals like humans, insects, and worms. This surprising discovery implies that the blueprint for forming a back-to-belly body axis could date back over 600 million years, to a common ancestor of cnidarians and bilaterians.
Biology
Unraveling Microtubule Mysteries: Scientists Crack Code on Cellular Scaffolding Secrets
Scientists found out how naturally unstable filaments decide whether to grow or to shorten.
Biotechnology
Unlocking the Cell’s Energy Hub: Scientists Reveal How ATP Enters the Endoplasmic Reticulum
A team of scientists has answered a long-standing question in cell biology, uncovering how the cell’s main energy currency, ATP, is transported into the endoplasmic reticulum (ER). Disrupted energy transport could affect diseases such as type 2 diabetes, cancer, and neurodegenerative disorders. The study confirms that the transporter protein SLC35B1 is the key gateway for ATP entry into the ER.
-
Detectors2 months ago
A New Horizon for Vision: How Gold Nanoparticles May Restore People’s Sight
-
Earth & Climate4 months ago
Retiring Abroad Can Be Lonely Business
-
Cancer3 months ago
Revolutionizing Quantum Communication: Direct Connections Between Multiple Processors
-
Agriculture and Food3 months ago
“A Sustainable Solution: Researchers Create Hybrid Cheese with 25% Pea Protein”
-
Diseases and Conditions4 months ago
Reducing Falls Among Elderly Women with Polypharmacy through Exercise Intervention
-
Earth & Climate3 months ago
Household Electricity Three Times More Expensive Than Upcoming ‘Eco-Friendly’ Aviation E-Fuels, Study Reveals
-
Albert Einstein4 months ago
Harnessing Water Waves: A Breakthrough in Controlling Floating Objects
-
Chemistry3 months ago
“Unveiling Hidden Patterns: A New Twist on Interference Phenomena”