While we try to keep things accurate, this content is part of an ongoing experiment and may not always be reliable.
Please double-check important details — we’re not responsible for how the information is used.
Biochemistry
A Breakthrough in Brain Research: The Iontronic Pipette Revolutionizes Neurological Studies
Researchers have developed a new type of pipette that can deliver ions to individual neurons without affecting the sensitive extracellular milieu. Controlling the concentration of different ions can provide important insights into how individual brain cells are affected, and how cells work together. The pipette could also be used for treatments.
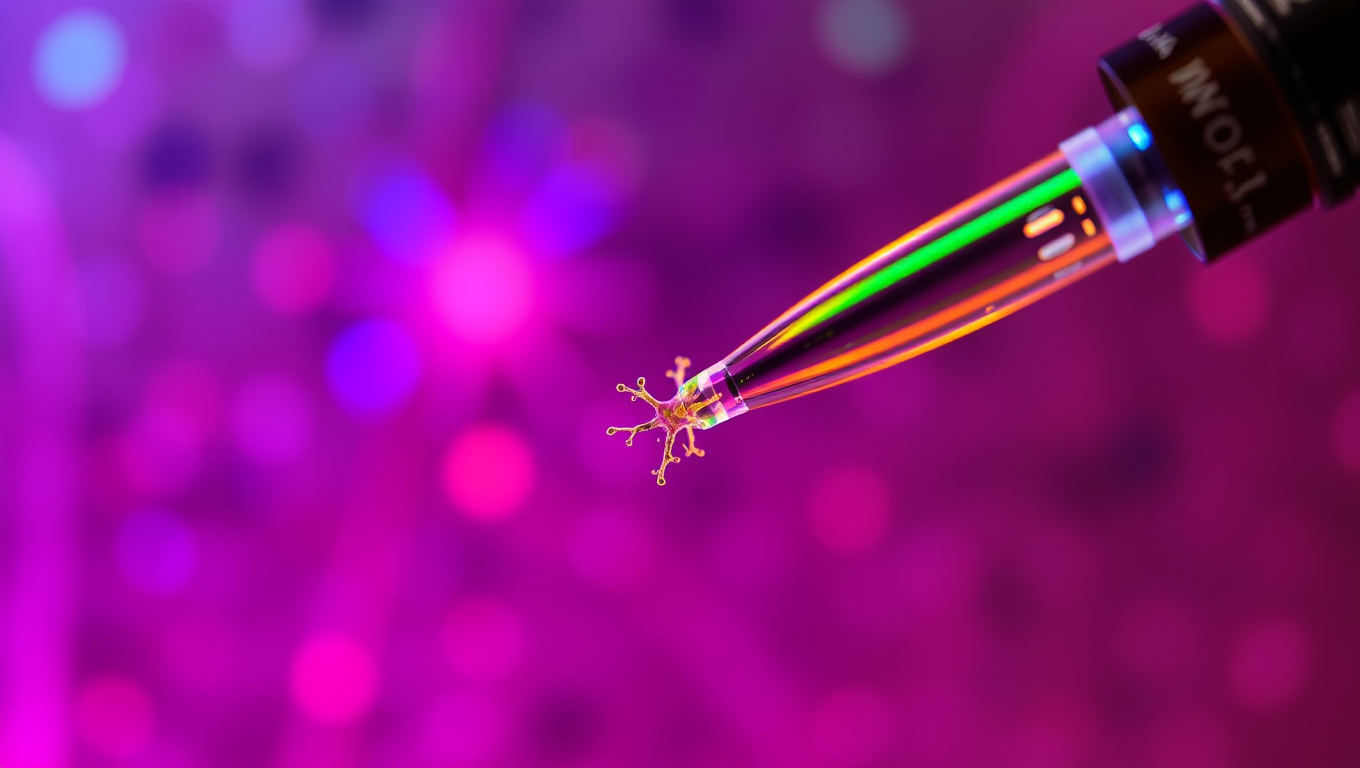
Biochemistry
Unveiling Molecular Motion: A Breakthrough in Synthetic Biology and Soft Matter Physics
Scientists have uncovered a previously unknown type of molecular motion inside DNA-based droplets: instead of spreading randomly, guest molecules advance in an organized wave. This surprising discovery opens the door to understanding how cells might organize internal processes without membranes. Using customizable DNA condensates as experimental models, the team showed how molecular waves emerge through precise DNA interactions. These insights could not only transform our grasp of cellular signaling but may even lay groundwork for treating neurodegenerative diseases by influencing how molecules behave inside aging cells.
Biochemistry
A Game-Changing mRNA Vaccine that’s More Effective and Less Costly to Develop
A new type of mRNA vaccine is more scalable and adaptable to continuously evolving viruses such as SARS-CoV-2 and H5N1, according to a new study.
Aerospace
Unveiling the Molecular Link Between Air Pollution and Pregnancy Risks: A Groundbreaking Study
A new study found exposure to specific tiny particles in air pollution during pregnancy are associated with increased risk of various negative birth outcomes.
-
Detectors3 months ago
A New Horizon for Vision: How Gold Nanoparticles May Restore People’s Sight
-
Earth & Climate4 months ago
Retiring Abroad Can Be Lonely Business
-
Cancer3 months ago
Revolutionizing Quantum Communication: Direct Connections Between Multiple Processors
-
Agriculture and Food3 months ago
“A Sustainable Solution: Researchers Create Hybrid Cheese with 25% Pea Protein”
-
Diseases and Conditions4 months ago
Reducing Falls Among Elderly Women with Polypharmacy through Exercise Intervention
-
Chemistry3 months ago
“Unveiling Hidden Patterns: A New Twist on Interference Phenomena”
-
Earth & Climate3 months ago
Household Electricity Three Times More Expensive Than Upcoming ‘Eco-Friendly’ Aviation E-Fuels, Study Reveals
-
Albert Einstein4 months ago
Harnessing Water Waves: A Breakthrough in Controlling Floating Objects