While we try to keep things accurate, this content is part of an ongoing experiment and may not always be reliable.
Please double-check important details — we’re not responsible for how the information is used.
Biochemistry Research
Unveiling Mammoth Diversity: A Glimpse into Their Evolutionary History
A new genomic study has uncovered long-lost genetic diversity in mammoth lineages spanning over a million years, providing new insights into the evolutionary history of these animals.
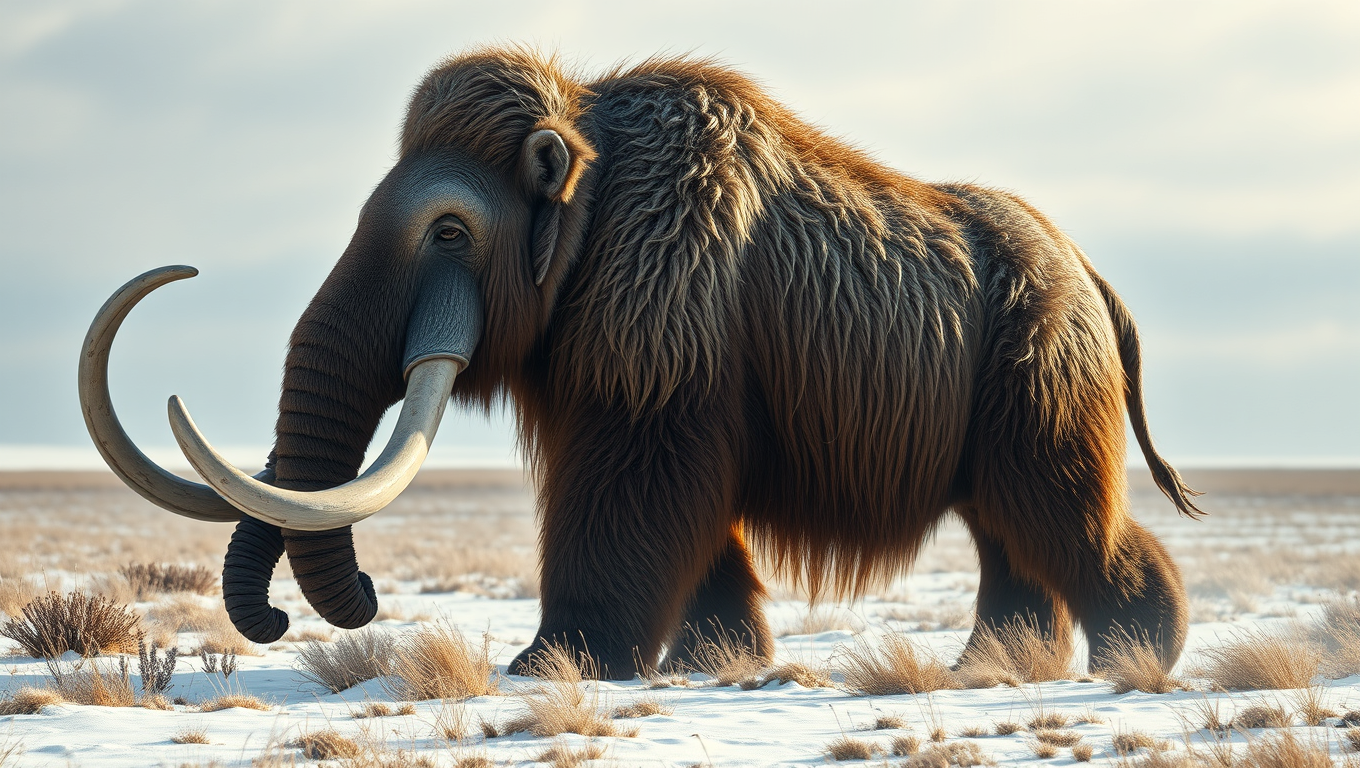
Biochemistry Research
The Whispering Womb: Uncovering the Secret Language of Embryonic Cells
Scientists found that embryonic skin cells “whisper” through faint mechanical tugs, using the same force-sensing proteins that make our ears ultrasensitive. By syncing these micro-movements, the cells choreograph the embryo’s shape, a dance captured with AI-powered imaging and computer models. Blocking the cells’ ability to feel the whispers stalls development, hinting that life’s first instructions are mechanical. The discovery suggests hearing hijacked an ancient force-sensing toolkit originally meant for building bodies.
Biochemistry Research
Unlocking the Secrets of Life: A Spontaneous Reaction that Could Have Started it All
Scientists have uncovered a surprising new way that urea—an essential building block for life—could have formed on the early Earth. Instead of requiring high temperatures or complex catalysts, this process occurs naturally on the surface of tiny water droplets like those in sea spray or fog. At this boundary between air and water, a unique chemical environment allows carbon dioxide and ammonia to combine and spontaneously produce urea, without any added energy. The finding offers a compelling clue in the mystery of life’s origins and hints that nature may have used simple, everyday phenomena to spark complex biological chemistry.
Biochemistry Research
The Double Edge of Love and War: How Female Earwigs Evolved Deadly Claws for Mate Competition
Female earwigs may be evolving exaggerated weaponry just like males. A study from Toho University found that female forceps, once assumed to be passive tools, show the same kind of outsized growth linked to sexual selection as the male’s iconic pincers. This means that female earwigs might be fighting for mates too specifically for access to non-aggressive males challenging long-standing assumptions in evolutionary biology.
-
Detectors3 months ago
A New Horizon for Vision: How Gold Nanoparticles May Restore People’s Sight
-
Earth & Climate4 months ago
Retiring Abroad Can Be Lonely Business
-
Cancer4 months ago
Revolutionizing Quantum Communication: Direct Connections Between Multiple Processors
-
Agriculture and Food4 months ago
“A Sustainable Solution: Researchers Create Hybrid Cheese with 25% Pea Protein”
-
Diseases and Conditions4 months ago
Reducing Falls Among Elderly Women with Polypharmacy through Exercise Intervention
-
Albert Einstein4 months ago
Harnessing Water Waves: A Breakthrough in Controlling Floating Objects
-
Chemistry4 months ago
“Unveiling Hidden Patterns: A New Twist on Interference Phenomena”
-
Earth & Climate4 months ago
Household Electricity Three Times More Expensive Than Upcoming ‘Eco-Friendly’ Aviation E-Fuels, Study Reveals