Biochemistry
Photonic Computing Needs More Nonlinearity: Acoustics Can Help
Neural networks are one typical structure on which artificial intelligence can be based. The term neural describes their learning ability, which to some extent mimics the functioning of neurons in our brains. To be able to work, several key ingredients are required: one of them is an activation function which introduces nonlinearity into the structure. A photonic activation function has important advantages for the implementation of optical neural networks based on light propagation. Researchers have now experimentally shown an all-optically controlled activation function based on traveling sound waves. It is suitable for a wide range of optical neural network approaches and allows operation in the so-called synthetic frequency dimension.
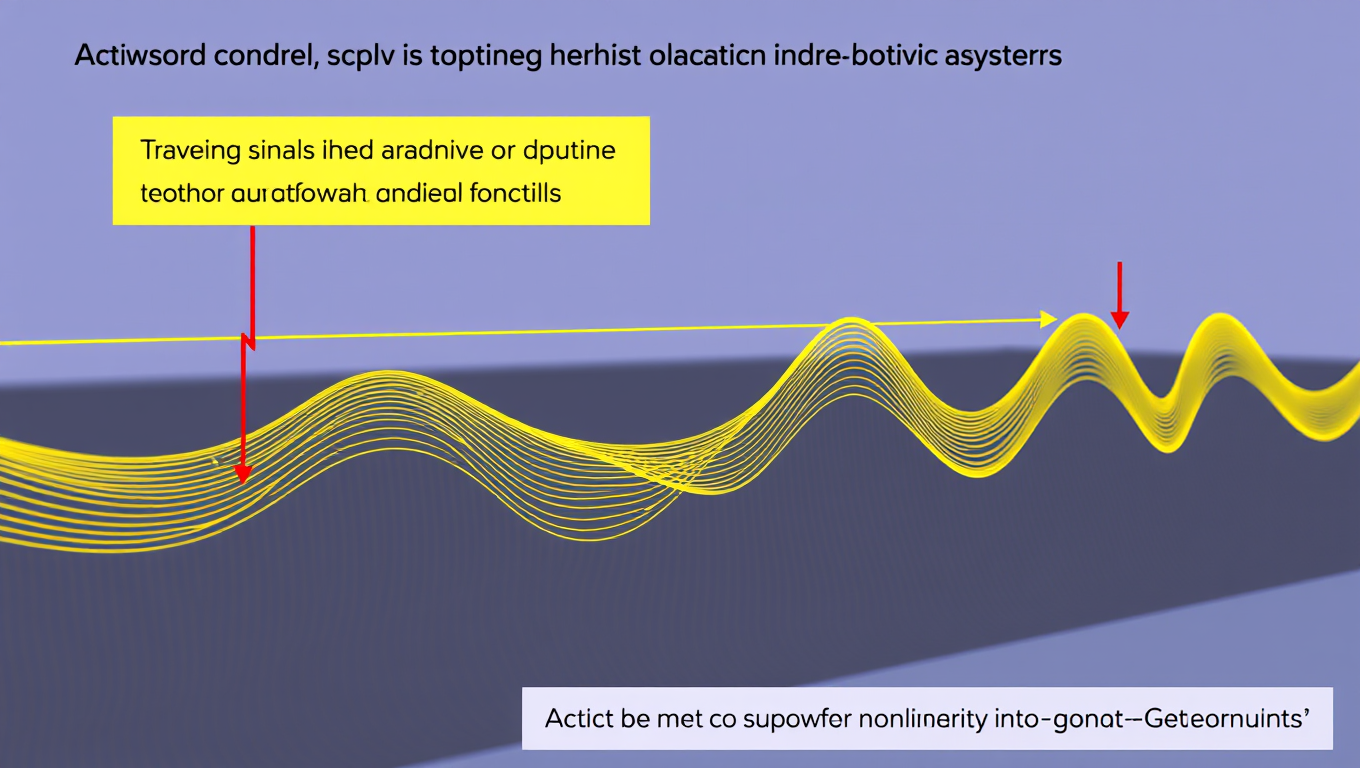
Batteries
“Unlocking the Secrets of Influenza Viruses: How Scientists Are Studying the Interaction between Viruses and Host Cells”
Influenza viruses are among the most likely triggers of future pandemics. A research team has developed a method that can be used to study the interaction of viruses with host cells in unprecedented detail. With the help of their new development, they have also analyzed how novel influenza viruses use alternative receptors to enter target cells.
Biochemistry
A Breakthrough in Brain Research: The Iontronic Pipette Revolutionizes Neurological Studies
Researchers have developed a new type of pipette that can deliver ions to individual neurons without affecting the sensitive extracellular milieu. Controlling the concentration of different ions can provide important insights into how individual brain cells are affected, and how cells work together. The pipette could also be used for treatments.
Biochemistry
Unraveling Nature’s Secret: How Velvet Worm Slime Could Revolutionize Sustainable Materials Design
A new discovery about the slime ejected by velvet worms could revolutionize sustainable material design. The findings outline how a naturally occurring protein structure, conserved across species from Australia, Singapore and Barbados over nearly 400 million years of evolution, enables the slime’s transformation from liquid to fiber and back again. It’s a discovery that could inspire next-generation recyclable bioplastics.
-
Detectors3 weeks ago
A New Horizon for Vision: How Gold Nanoparticles May Restore People’s Sight
-
Earth & Climate2 months ago
Retiring Abroad Can Be Lonely Business
-
Cancer2 months ago
Revolutionizing Quantum Communication: Direct Connections Between Multiple Processors
-
Agriculture and Food2 months ago
“A Sustainable Solution: Researchers Create Hybrid Cheese with 25% Pea Protein”
-
Diseases and Conditions2 months ago
Reducing Falls Among Elderly Women with Polypharmacy through Exercise Intervention
-
Albert Einstein2 months ago
Harnessing Water Waves: A Breakthrough in Controlling Floating Objects
-
Alternative Fuels2 months ago
Unlocking the Secrets of Supercritical Water: A New Understanding of Its Structure
-
Earth & Climate2 months ago
Household Electricity Three Times More Expensive Than Upcoming ‘Eco-Friendly’ Aviation E-Fuels, Study Reveals