While we try to keep things accurate, this content is part of an ongoing experiment and may not always be reliable.
Please double-check important details — we’re not responsible for how the information is used.
Alternative Fuels
“Beyond Conventional Thinking: Unlocking the Potential of Single Atom Catalysts with Hydrogen Binding Energy”
Conventional thinking holds that the metal site in single atom catalysts (SACs) has been a limiting factor to the continued improvement of the design and, therefore, the continued improvement of the capability of these SACs. More specifically, the lack of outside-the-box thinking when it comes to the crucial hydrogen evolution reaction (HER), a half-reaction resulting in the splitting of water, has contributed to a lack of advancement in this field. New research emphasizes the importance of pushing the limits of the metal site design in SACs to optimize the HER and addressing the poisoning effects of HO* and O* that might affect the reaction. All of these improvements could lead to an improved performance of the reaction, which can make sustainable energy storage or hydrogen production more available.
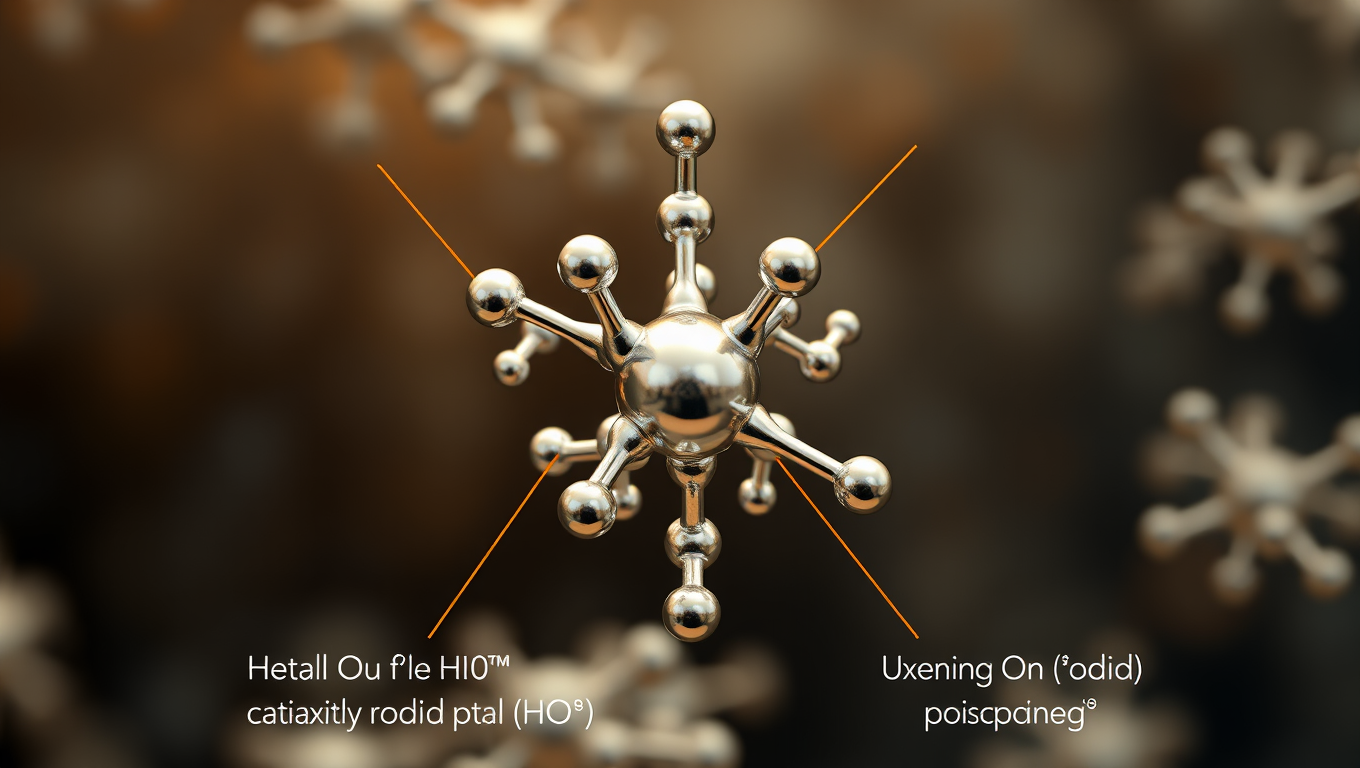
Alternative Fuels
Breaking Ground in Green Energy: An Iron Oxide Catalyst for Efficient Hydrogen Production
As the world shifts toward sustainable energy sources, ‘green hydrogen’ – hydrogen produced without emitting carbon – has emerged as a leading candidate for clean power. Scientists have now developed a new iron-based catalyst that more than doubles the conversion efficiency of thermochemical green hydrogen production.
Alternative Fuels
EV Battery Recycling Key to Future Lithium Supplies
Lightweight, powerful lithium-ion batteries are crucial for the transition to electric vehicles, and global demand for lithium is set to grow rapidly over the next 25 years. A new analysis looks at how new mining operations and battery recycling could meet that demand. Recycling could play a big role in easing supply constraints, the researchers found.
Alternative Fuels
Unveiling Electron Secrets: A Groundbreaking Experiment on the Bound Electron g-Factor in Lithium-Like Tin
Researchers present new experimental and theoretical results for the bound electron g-factor in lithium-like tin which has a much higher nuclear charge than any previous measurement. The experimental accuracy reached a level of 0.5 parts per billion. Using an enhanced interelectronic QED method, the theoretical prediction for the g-factor reached a precision of 6 parts per billion.
-
Detectors2 months ago
A New Horizon for Vision: How Gold Nanoparticles May Restore People’s Sight
-
Earth & Climate4 months ago
Retiring Abroad Can Be Lonely Business
-
Cancer3 months ago
Revolutionizing Quantum Communication: Direct Connections Between Multiple Processors
-
Agriculture and Food3 months ago
“A Sustainable Solution: Researchers Create Hybrid Cheese with 25% Pea Protein”
-
Diseases and Conditions4 months ago
Reducing Falls Among Elderly Women with Polypharmacy through Exercise Intervention
-
Earth & Climate3 months ago
Household Electricity Three Times More Expensive Than Upcoming ‘Eco-Friendly’ Aviation E-Fuels, Study Reveals
-
Chemistry3 months ago
“Unveiling Hidden Patterns: A New Twist on Interference Phenomena”
-
Albert Einstein4 months ago
Harnessing Water Waves: A Breakthrough in Controlling Floating Objects