Biochemistry Research
Bringing Balance to Genetics Education: Why We Need to Teach Eugenics in College Curriculum
To encourage scientists to speak up when people misuse science to serve political agendas, biology professor Mark Peifer of the University of North Carolina at Chapel Hill argues that eugenics should be included in college genetics curriculums.
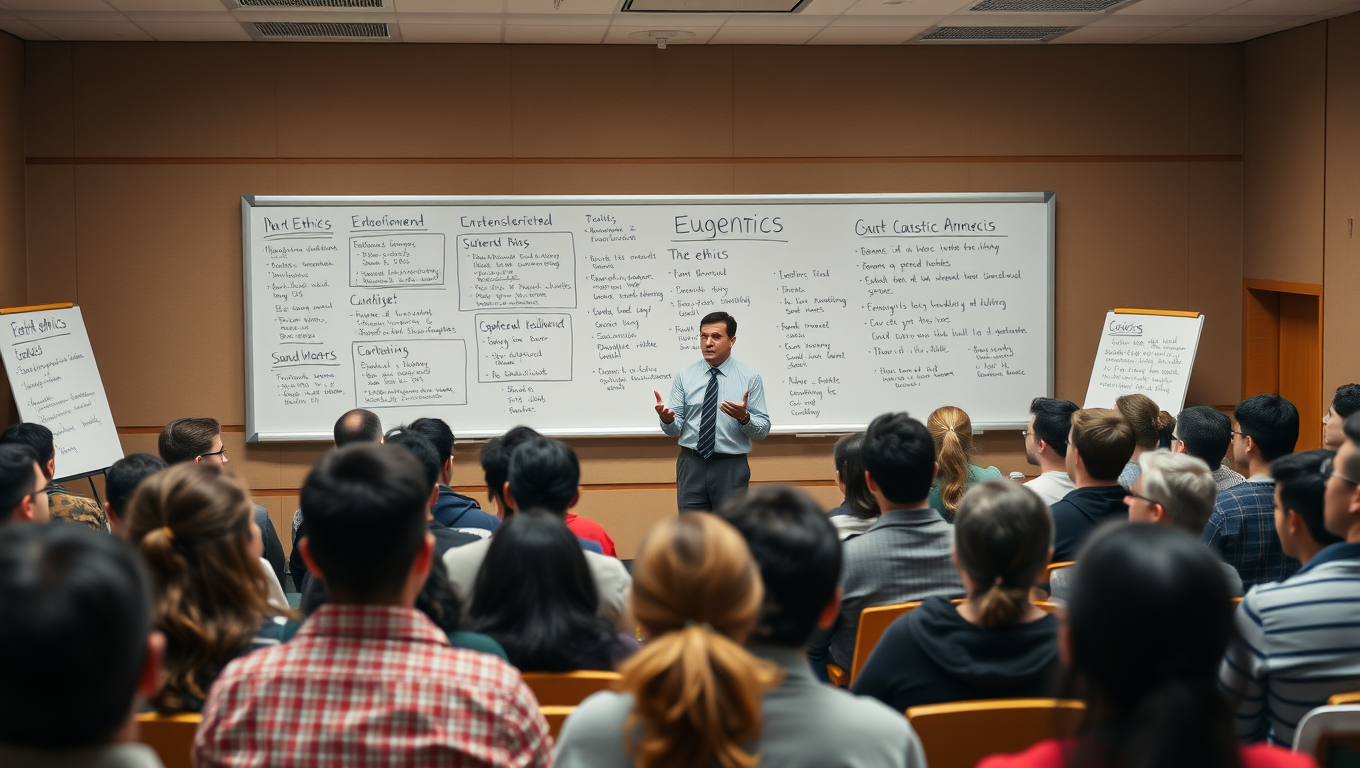
Bacteria
Unlocking Efficiency: Researchers Reveal Secrets of Cell Division with Min Proteins
The Min protein system prevents abnormal cell division in bacteria, but is poorly understood. Researchers have uncovered how engineered e.coli bacteria control protein levels for maximum efficiency.
Biochemistry Research
Unlocking Cell Movement: Researchers Crack the Code on How Cells Travel Through the Body
Scientists have discovered how chemokines and G protein-coupled receptors selectively bind each other to control how cells move.
Biochemistry
“Tailoring Gene Editing with Machine Learning: A Breakthrough in CRISPR-Cas9 Enzyme Engineering”
Genome editing has advanced at a rapid pace with promising results for treating genetic conditions — but there is always room for improvement. A new paper showcases the power of scalable protein engineering combined with machine learning to boost progress in the field of gene and cell therapy. In their study, authors developed a machine learning algorithm — known as PAMmla — that can predict the properties of about 64 million genome editing enzymes. The work could help reduce off-target effects and improve editing safety, enhance editing efficiency, and enable researchers to predict customized enzymes for new therapeutic targets.
-
Detectors3 weeks ago
A New Horizon for Vision: How Gold Nanoparticles May Restore People’s Sight
-
Earth & Climate2 months ago
Retiring Abroad Can Be Lonely Business
-
Cancer2 months ago
Revolutionizing Quantum Communication: Direct Connections Between Multiple Processors
-
Agriculture and Food2 months ago
“A Sustainable Solution: Researchers Create Hybrid Cheese with 25% Pea Protein”
-
Diseases and Conditions2 months ago
Reducing Falls Among Elderly Women with Polypharmacy through Exercise Intervention
-
Albert Einstein2 months ago
Harnessing Water Waves: A Breakthrough in Controlling Floating Objects
-
Alternative Fuels2 months ago
Unlocking the Secrets of Supercritical Water: A New Understanding of Its Structure
-
Earth & Climate2 months ago
Household Electricity Three Times More Expensive Than Upcoming ‘Eco-Friendly’ Aviation E-Fuels, Study Reveals