Brain Injury
Uncovering the Secrets of Thirst and Hunger Neurons: A New Frontier in Brain Research
New research shines light on how the brain interprets nutritional and hydration needs and turns them into action.
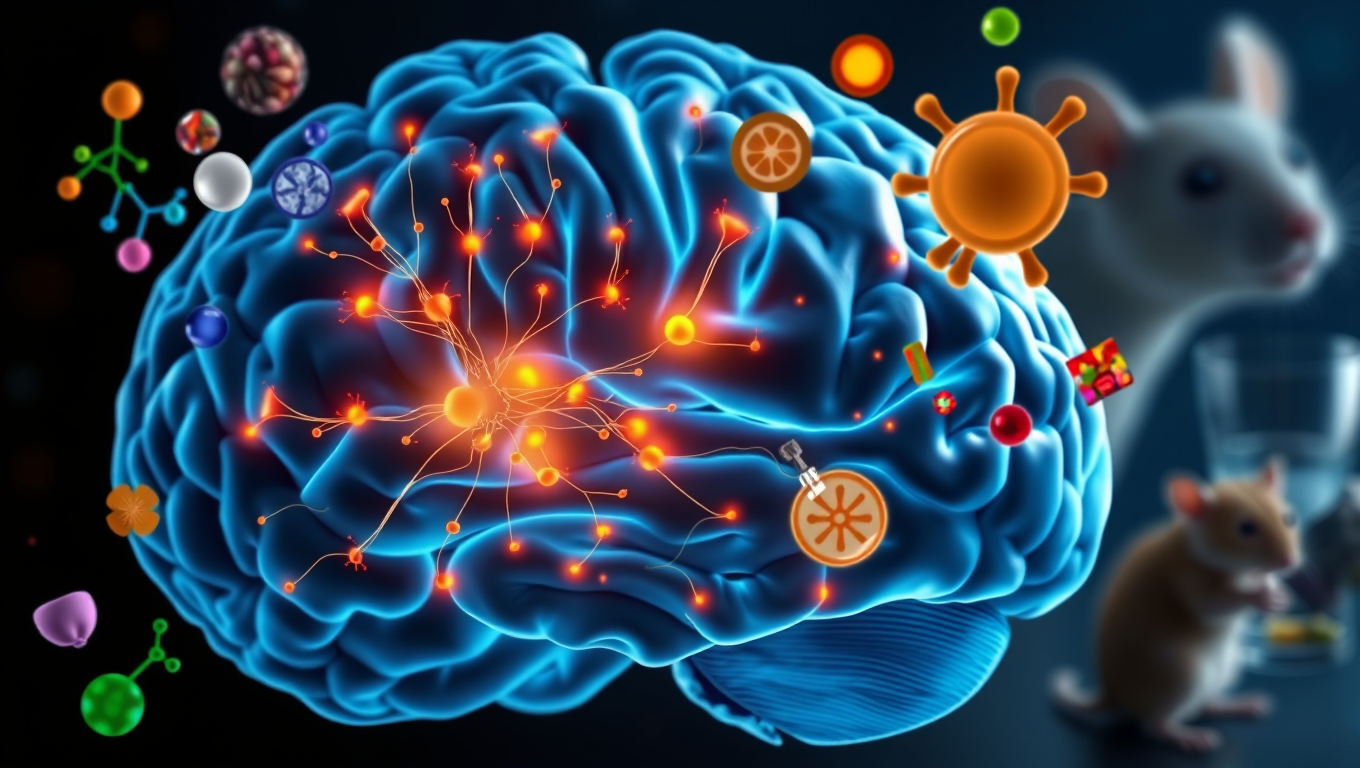
Brain Injury
“Resonating with Sound: Study Suggests Our Brains Physically Respond to Music”
Psychologists suggest our brains and bodies don’t just understand music, they physically resonate with it. These discoveries, based on findings in neuroscience, music, and psychology, support Neural Resonance Theory (NRT).
Behavior
The Adaptive Nature of Object Recognition: How Experience Shapes Our Perception of Reality
New findings suggest neurons have much more functional dexterity than scientists previously realized.
Brain Injury
Uncovering the Aggressive Nature of Glioblastoma: ZIP4’s Role in Brain Tumor Growth
Researchers detail their discoveries about why the brain tumor glioblastoma is so aggressive. Their findings center on ZIP4, a protein that transports zinc throughout the body and sets off a cascade of events that drive tumor growth.
-
Detectors3 weeks ago
A New Horizon for Vision: How Gold Nanoparticles May Restore People’s Sight
-
Earth & Climate2 months ago
Retiring Abroad Can Be Lonely Business
-
Cancer2 months ago
Revolutionizing Quantum Communication: Direct Connections Between Multiple Processors
-
Agriculture and Food2 months ago
“A Sustainable Solution: Researchers Create Hybrid Cheese with 25% Pea Protein”
-
Diseases and Conditions2 months ago
Reducing Falls Among Elderly Women with Polypharmacy through Exercise Intervention
-
Albert Einstein2 months ago
Harnessing Water Waves: A Breakthrough in Controlling Floating Objects
-
Alternative Fuels2 months ago
Unlocking the Secrets of Supercritical Water: A New Understanding of Its Structure
-
Earth & Climate1 month ago
Household Electricity Three Times More Expensive Than Upcoming ‘Eco-Friendly’ Aviation E-Fuels, Study Reveals