Disorders and Syndromes
“Placenta Plays Key Role in Genetic Risk for Schizophrenia and Other Neuropsychiatric Disorders”
An international team has identified associations between modifications in the placenta and the risk of developing schizophrenia, bipolar disorder, and major depression disorder.
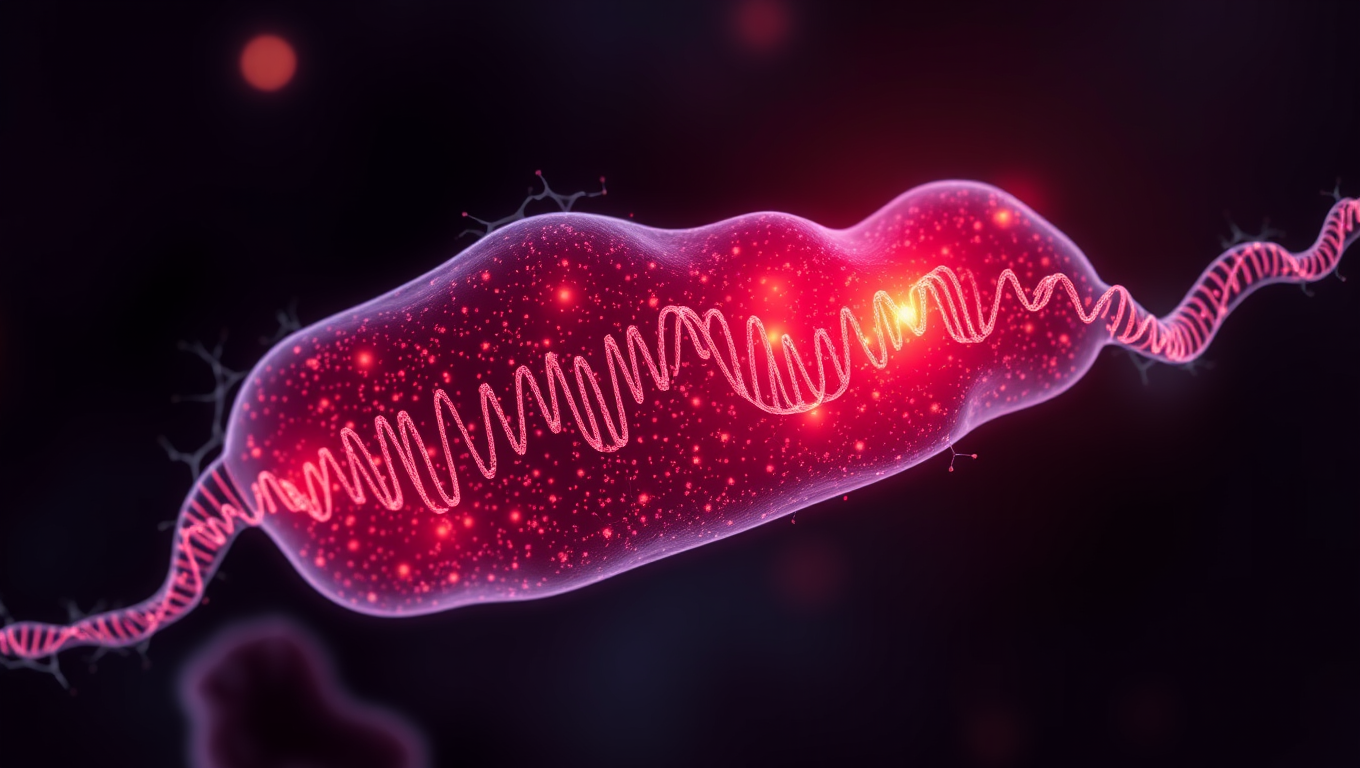
Biochemistry
A Breakthrough in Brain Research: The Iontronic Pipette Revolutionizes Neurological Studies
Researchers have developed a new type of pipette that can deliver ions to individual neurons without affecting the sensitive extracellular milieu. Controlling the concentration of different ions can provide important insights into how individual brain cells are affected, and how cells work together. The pipette could also be used for treatments.
Anxiety
Unveiling the Hidden Role of Dopamine: A Breakthrough in Understanding Anxiety and Depression
Researchers have discovered distinct roles for two dopamine receptors located on nerve cells within the portion of the brain that controls approach vs. avoidance behavior.
Diseases and Conditions
Unlocking the Secrets of Huntington’s Disease: Two Proteins with Opposing Effects
Researchers found that inhibiting GSK-3 led to less defects in the axonal transport process and less neuronal cell death, while inhibiting ERK1 led to more transport problems and more cell death.
-
Detectors3 weeks ago
A New Horizon for Vision: How Gold Nanoparticles May Restore People’s Sight
-
Earth & Climate2 months ago
Retiring Abroad Can Be Lonely Business
-
Cancer2 months ago
Revolutionizing Quantum Communication: Direct Connections Between Multiple Processors
-
Agriculture and Food2 months ago
“A Sustainable Solution: Researchers Create Hybrid Cheese with 25% Pea Protein”
-
Diseases and Conditions2 months ago
Reducing Falls Among Elderly Women with Polypharmacy through Exercise Intervention
-
Albert Einstein2 months ago
Harnessing Water Waves: A Breakthrough in Controlling Floating Objects
-
Alternative Fuels2 months ago
Unlocking the Secrets of Supercritical Water: A New Understanding of Its Structure
-
Chemistry1 month ago
“Unveiling Hidden Patterns: A New Twist on Interference Phenomena”