Batteries
A Safer Way Forward: Developing Miniature Batteries for Research and Testing
Overheating batteries are a serious risk, in the worst cases leading to fires and explosion. A team has now developed a simple, cost-effective method to test the safety of lithium-ion batteries, which opens up opportunities for research into new and safer batteries for the future. The researchers created an intentionally unstable battery which is more sensitive to changes that could cause overheating. The battery is one-fiftieth the size of conventional batteries, so is less resource intensive and tests can be carried out in a smaller lab environment.
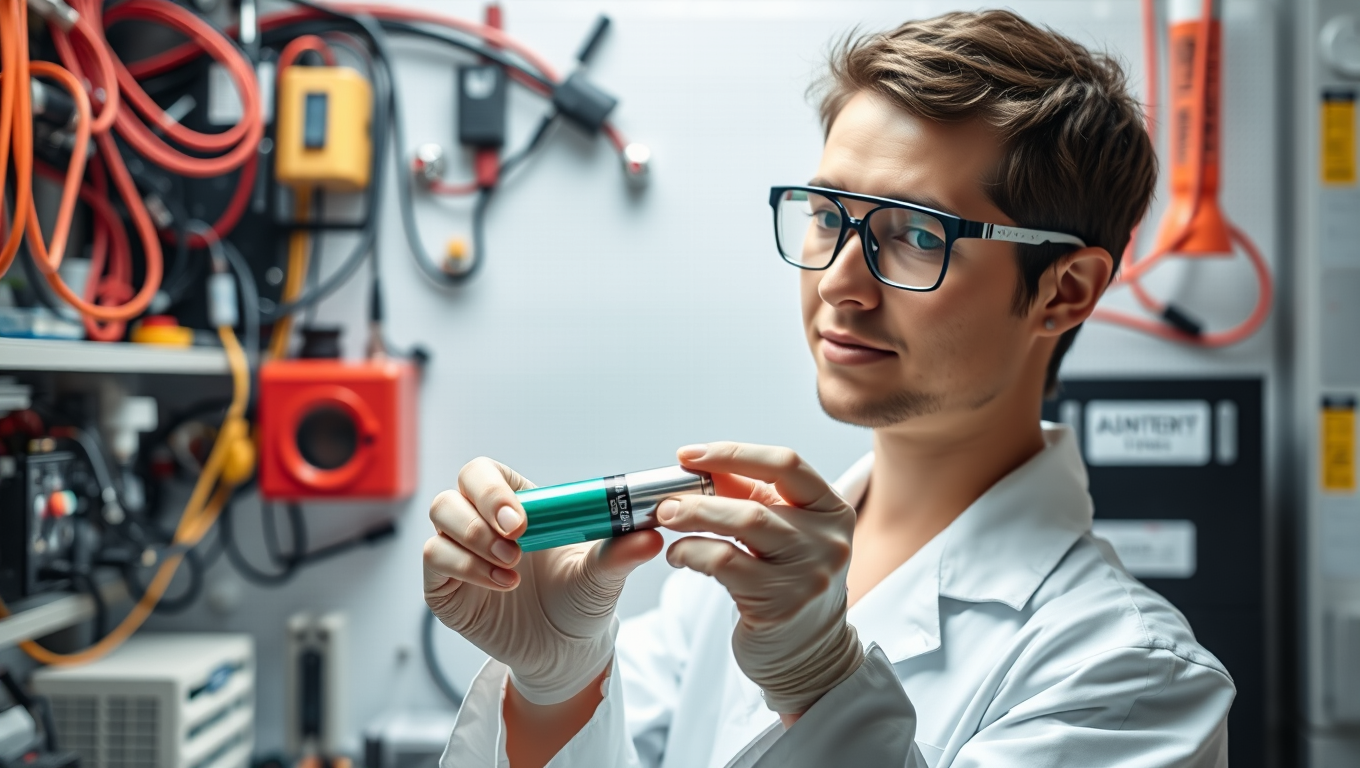
Batteries
“Unlocking the Secrets of Influenza Viruses: How Scientists Are Studying the Interaction between Viruses and Host Cells”
Influenza viruses are among the most likely triggers of future pandemics. A research team has developed a method that can be used to study the interaction of viruses with host cells in unprecedented detail. With the help of their new development, they have also analyzed how novel influenza viruses use alternative receptors to enter target cells.
Alternative Fuels
Breakthrough in Fuel Cell Lifespan: Paving the Way for Clean Long-Haul Trucking
Researchers have developed a new catalyst design capable of pushing the projected fuel cell catalyst lifespans to 200,000 hours. The research marks a significant step toward the widespread adoption of fuel cell technology in heavy-duty vehicles, such as long-haul tractor trailers. While platinum-alloy catalysts have historically delivered superior chemical reactions, the alloying elements leach out over time, diminishing catalytic performance. The degradation is further accelerated by the demanding voltage cycles required to power heavy-duty vehicles. To address this challenge, the team has engineered a durable catalyst architecture with a novel design that shields platinum from the degradation typically observed in alloy systems.
Alternative Fuels
Unlocking Safe and Efficient Fast-Charging Batteries with a New Computational Model
An innovative new computational model developed by an engineer could lead to fast-charging lithium-ion batteries that are safer and longer-lasting.
-
Detectors3 weeks ago
A New Horizon for Vision: How Gold Nanoparticles May Restore People’s Sight
-
Earth & Climate2 months ago
Retiring Abroad Can Be Lonely Business
-
Cancer2 months ago
Revolutionizing Quantum Communication: Direct Connections Between Multiple Processors
-
Agriculture and Food2 months ago
“A Sustainable Solution: Researchers Create Hybrid Cheese with 25% Pea Protein”
-
Diseases and Conditions2 months ago
Reducing Falls Among Elderly Women with Polypharmacy through Exercise Intervention
-
Albert Einstein2 months ago
Harnessing Water Waves: A Breakthrough in Controlling Floating Objects
-
Alternative Fuels2 months ago
Unlocking the Secrets of Supercritical Water: A New Understanding of Its Structure
-
Earth & Climate2 months ago
Household Electricity Three Times More Expensive Than Upcoming ‘Eco-Friendly’ Aviation E-Fuels, Study Reveals