While we try to keep things accurate, this content is part of an ongoing experiment and may not always be reliable.
Please double-check important details — we’re not responsible for how the information is used.
Energy and Resources
Metamaterial Marvels: Highly Twisted Rods Unlock Large Energy Storage Potential
An international research team has developed mechanical metamaterials with a high elastic energy density. Highly twisted rods that deform helically provide these metamaterials with a high stiffness and enable them to absorb and release large amounts of elastic energy. The researchers conducted simple compression experiments to confirm the initial theoretical results.
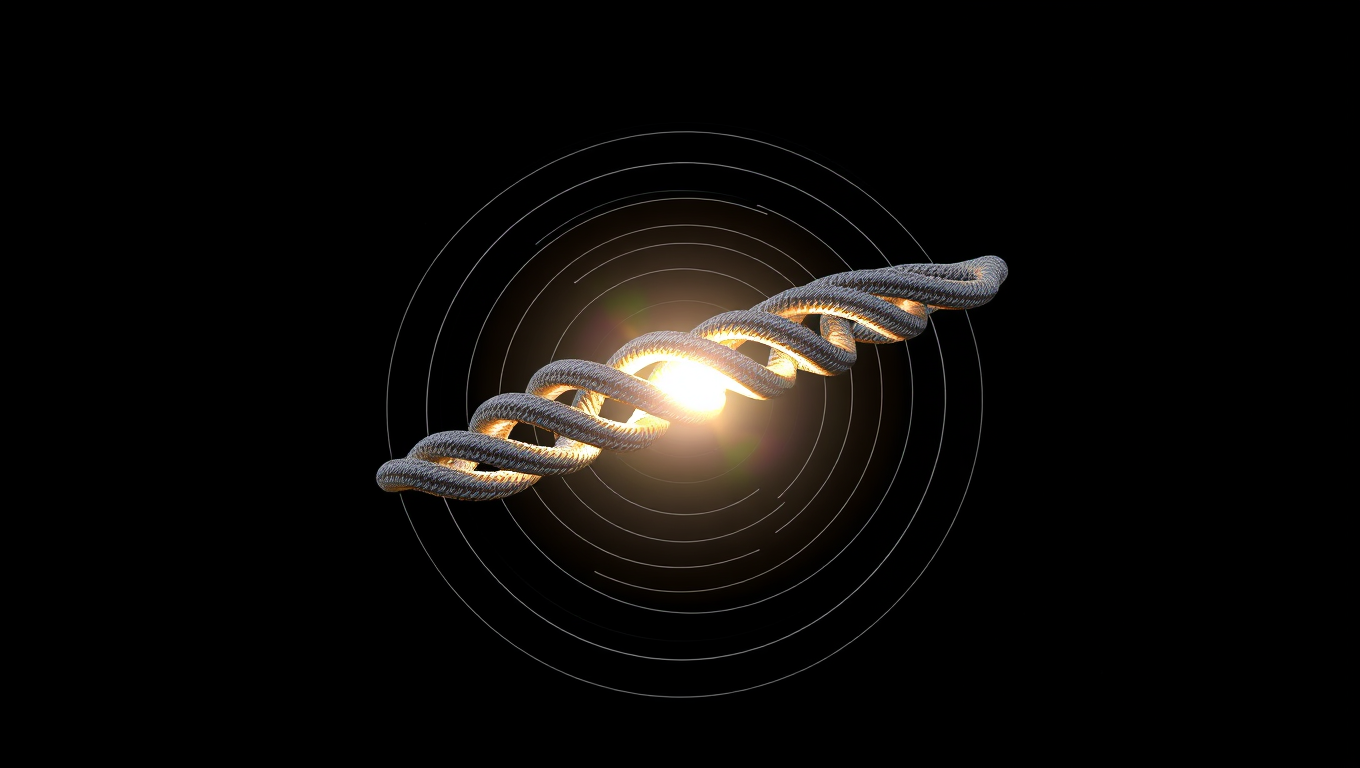
Chemistry
Defying Physics: Atacamite’s Rare Magnetic Cooling Property
Deep in Chile’s Atacama Desert, scientists studied a green crystal called atacamite—and discovered it can cool itself dramatically when placed in a magnetic field. Unlike a regular fridge, this effect doesn’t rely on gases or compressors. Instead, it’s tied to the crystal’s unusual inner structure, where tiny magnetic forces get tangled in a kind of “frustration.” When those tangled forces are disrupted by magnetism, the crystal suddenly drops in temperature. It’s a strange, natural trick that could someday help us build greener, more efficient ways to cool things.
Automotive and Transportation
A Breakthrough in Ammonia Production: Harnessing Human-Made Lightning for Sustainable Fertilizers
Australian scientists have discovered a method to produce ammonia—an essential component in fertilizers—using only air and electricity. By mimicking lightning and channeling that energy through a small device, they’ve bypassed the traditional, fossil fuel-heavy method that’s been used for over a century. This breakthrough could lead to cleaner, cheaper fertilizer and even help power the future, offering a potential alternative fuel source for industries like shipping.
Chemistry
Mapping Platinum Atoms for a Greener Future in Catalysis
A precious metal used everywhere from car exhaust systems to fuel cells, platinum is an incredibly efficient catalyst—but it’s costly and carbon-intensive. Now, a serendipitous collaboration between scientists at ETH Zurich and other European institutions has opened a new frontier in understanding and optimizing platinum-based catalysts at the atomic level.
-
Detectors3 months ago
A New Horizon for Vision: How Gold Nanoparticles May Restore People’s Sight
-
Earth & Climate4 months ago
Retiring Abroad Can Be Lonely Business
-
Cancer4 months ago
Revolutionizing Quantum Communication: Direct Connections Between Multiple Processors
-
Agriculture and Food4 months ago
“A Sustainable Solution: Researchers Create Hybrid Cheese with 25% Pea Protein”
-
Diseases and Conditions4 months ago
Reducing Falls Among Elderly Women with Polypharmacy through Exercise Intervention
-
Albert Einstein4 months ago
Harnessing Water Waves: A Breakthrough in Controlling Floating Objects
-
Earth & Climate4 months ago
Household Electricity Three Times More Expensive Than Upcoming ‘Eco-Friendly’ Aviation E-Fuels, Study Reveals
-
Chemistry3 months ago
“Unveiling Hidden Patterns: A New Twist on Interference Phenomena”