Civil Engineering
Unraveling the Secrets of Dendritic Growth: AI Reveals Hidden Patterns in Thin Films
Dendritic structures that emerge during the growth of thin films are a major obstacle in large-area fabrication, a key step towards commercialization. However, current methods of studying dendrites involve crude visual inspection and subjective analysis. Moreover, growth optimization methods for controlling dendrite formation require extensive trial and error. Now, researchers have developed a new AI model that incorporates topology analysis and free energy to reveal the specific conditions and mechanisms that drive dendrite branching.
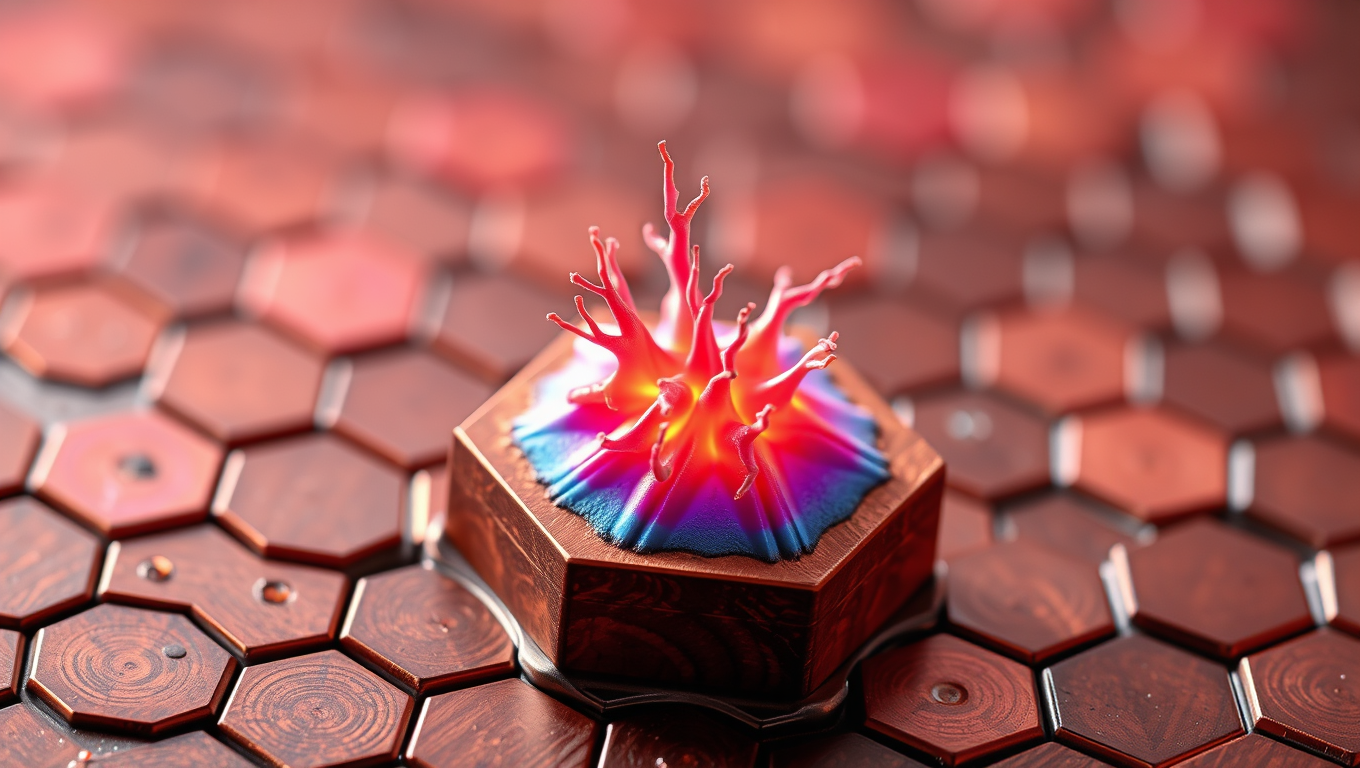
Artificial Intelligence
The Hidden Barrier to Advanced Robotic Touch
Researchers argue that the problem that has been lurking in the margins of many papers about touch sensors lies in the robotic skin itself.
Aerospace
“Challenging the Classics: Researchers Reveal New Insights into Material Deformation under Stress”
Scientists have expanded on a longstanding model governing the mechanics behind slip banding, a process that produces strain marks in metals under compression, gaining a new understanding of the behavior of advanced materials critical to energy systems, space exploration and nuclear applications.
Biochemistry
Unveiling the Mystery of Crystals: Scientists Discover a New Type and Shed Light on Their Formation
Crystals — from sugar and table salt to snowflakes and diamonds — don’t always grow in a straightforward way. Researchers have now captured this journey from amorphous blob to orderly structures. In exploring how crystals form, the researchers also came across an unusual, rod-shaped crystal that hadn’t been identified before, naming it ‘Zangenite’ for the graduate student who discovered it.
-
Detectors3 weeks ago
A New Horizon for Vision: How Gold Nanoparticles May Restore People’s Sight
-
Earth & Climate2 months ago
Retiring Abroad Can Be Lonely Business
-
Cancer2 months ago
Revolutionizing Quantum Communication: Direct Connections Between Multiple Processors
-
Agriculture and Food2 months ago
“A Sustainable Solution: Researchers Create Hybrid Cheese with 25% Pea Protein”
-
Diseases and Conditions2 months ago
Reducing Falls Among Elderly Women with Polypharmacy through Exercise Intervention
-
Albert Einstein2 months ago
Harnessing Water Waves: A Breakthrough in Controlling Floating Objects
-
Alternative Fuels2 months ago
Unlocking the Secrets of Supercritical Water: A New Understanding of Its Structure
-
Earth & Climate2 months ago
Household Electricity Three Times More Expensive Than Upcoming ‘Eco-Friendly’ Aviation E-Fuels, Study Reveals