Early Climate
Unveiling the Ancient Secrets of the Pacific Ocean: A Breakthrough in Understanding Earth’s Volcanic History
A new study traces a 120-million-year-old ‘super-eruption’ to its source, offering new insights into Earth’s complex geological history.
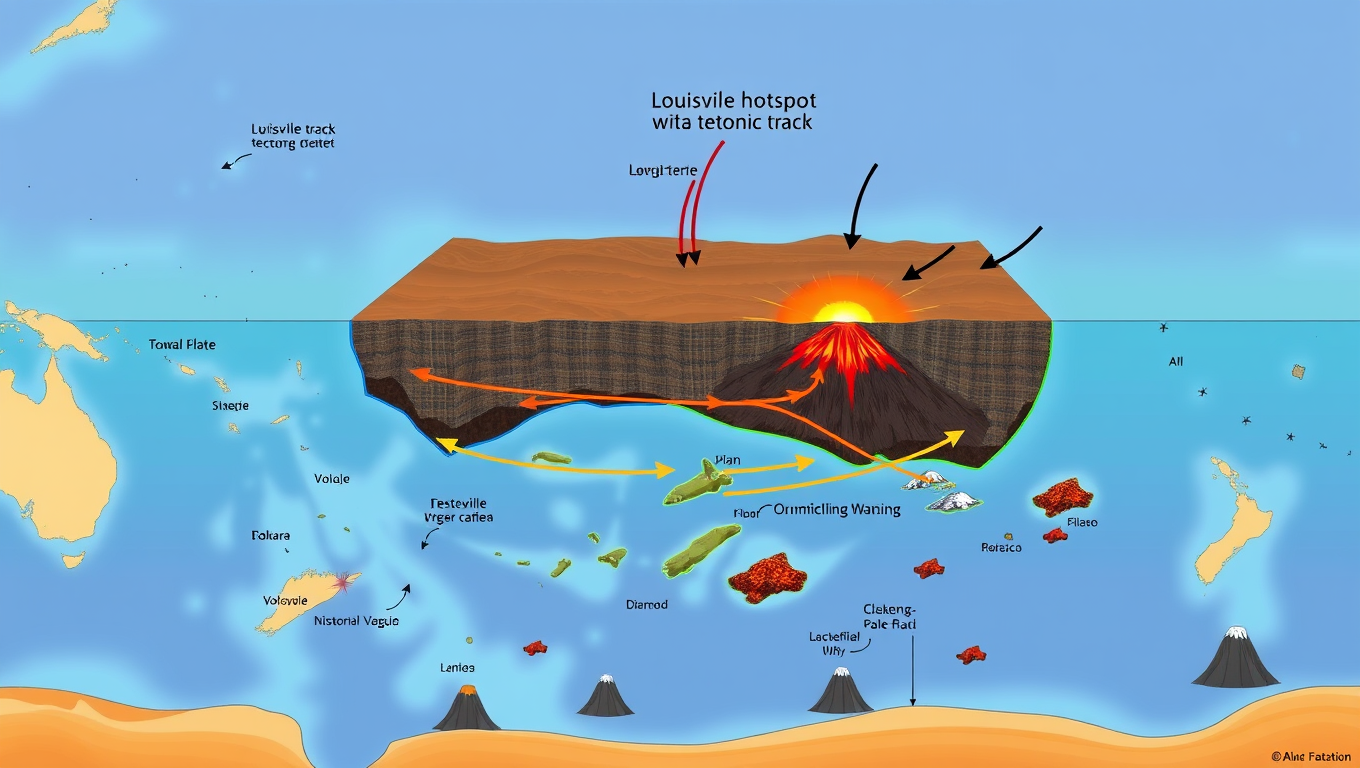
Anthropology
Uncovering the Secrets of Utah’s Wasatch Fault: A Study on Earthquake Vulnerability
Using rock samples collected from the Wasatch Fault, geoscientists combined experiments and analysis with examinations of fault rock textures. The team’s research revealed significant clues about the Wasatch Fault’s earthquake risk. Researchers explain why properties of fault rocks and geologic events that occurred more than a billion years ago portend worrisome seismic activity for Utah’s population center.
Archaeology
The Hidden World of Mountain Streamflow: A Surprise from the West’s Snow-Capped Mountains
Hydrologists show most streamflow out of the West’s mountains is old snowmelt on a multi-year underground journey. New study finds that spring runoff is on average 5 years old.
Early Climate
A Shift in Oceanic Top Predators: Uncovering the Mystery of Mid-Cretaceous Marine Extinctions
The highest trophic niches in Mesozoic oceans were filled by diverse marine reptiles, including ichthyosaurians, plesiosaurians, and thalattosuchians, dominating food webs during the Jurassic and Early Cretaceous. Yet during the mid-Cretaceous, ichthyosaurs, thalattosuchians, and pliosaurids vanished, replaced by mosasaurs, xenopsarian plesiosaurians, and new groups like sharks, fish, turtles, and birds. This shift restructured marine ecosystems.
-
Detectors3 weeks ago
A New Horizon for Vision: How Gold Nanoparticles May Restore People’s Sight
-
Earth & Climate2 months ago
Retiring Abroad Can Be Lonely Business
-
Cancer2 months ago
Revolutionizing Quantum Communication: Direct Connections Between Multiple Processors
-
Agriculture and Food2 months ago
“A Sustainable Solution: Researchers Create Hybrid Cheese with 25% Pea Protein”
-
Diseases and Conditions2 months ago
Reducing Falls Among Elderly Women with Polypharmacy through Exercise Intervention
-
Albert Einstein2 months ago
Harnessing Water Waves: A Breakthrough in Controlling Floating Objects
-
Alternative Fuels2 months ago
Unlocking the Secrets of Supercritical Water: A New Understanding of Its Structure
-
Chemistry1 month ago
“Unveiling Hidden Patterns: A New Twist on Interference Phenomena”